Ready for an uplifting story? Stage 18 of the Tour de France skirts around the southern edge of the magnificent Parc National des Écrins, a mountainous massif with peaks reaching 4102 meters of elevation. The compressive forces driving the formation of these mountains ended millions of years ago. Yet, they are still rising by around two millimetres each year. This means that since the first Tour de France in 1903, the mountains north of Stage 18 may have risen by as much as 240 millimetres.
In this uplifting story, we explore the processes driving uplift in that part of the Alps and ask ourselves: if the collision is dead, why are the Western Alps actively rising?
How do we know the Alps are rising?
Though it may seem stationary, much of Earth’s surface is slowly on the move. Geoscientists track these movements using the same GPS technology as your smartphone. The GPS triangulates your position to within a few meters using signals from a constellation of satellites. Modern high-end GPS systems boast incredible accuracy, capable of pinpointing locations with millimetre precision. By continuously monitoring fixed GPS stations over many years, geoscientists measure shifting tectonic plates and vertical changes in elevation. Tomorrow, on stage 19, we tell you more about how this works.
GPS measurements reveal about one to three millimetres a year of uplift along the length of the Alps. This may seem slow, but over the vastness of geological time, uplift profoundly shapes landscapes. It builds topography, feeds erosion, redirects rivers and coastlines, and affects stresses in the crust that drive seismicity.
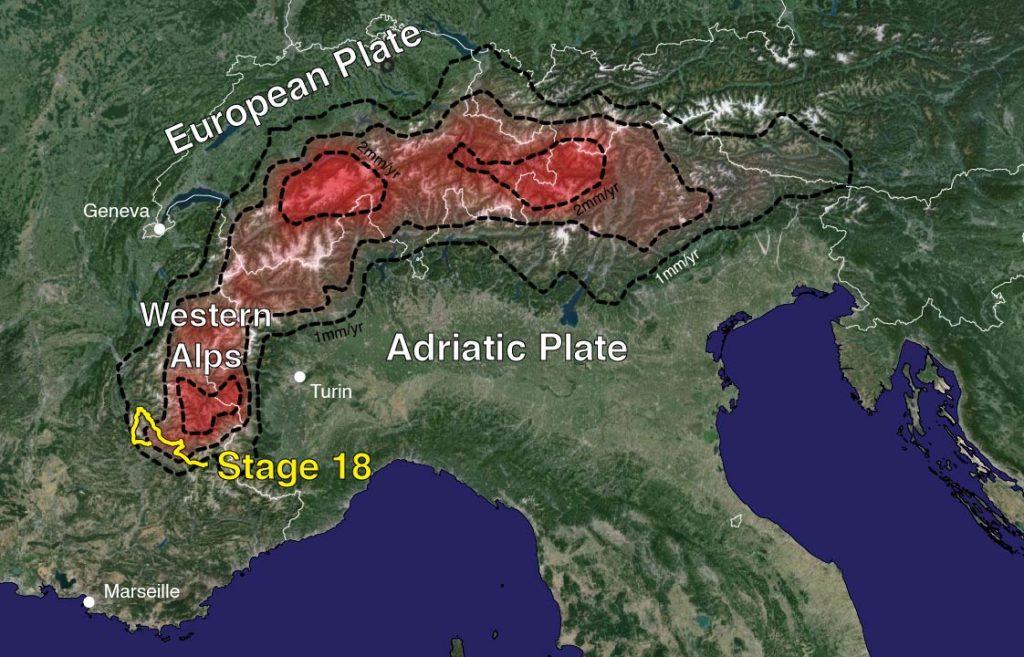
High topograhy
To explain the rise of the Western Alps, we need to jump back a step. Have you ever considered why mountain belts are higher than their surroundings? Earth’s outer layer—the lithosphere—comprises the crust and lithospheric mantle. The lithosphere floats on the denser semi-fluid asthenospheric mantle below, just as ice floats in water. The top of an iceberg is emergent, supported by the buoyancy of the submerged ice. The tip of the iceberg will rise higher if supported by a thicker, larger volume of submerged ice.
Earth’s topographic diversity, from the depths of the oceans to the heights of mountains, is primarily due to differences in the thickness of the Earth’s crust. That is the less dense upper layer of the lithosphere. Beneath the oceans, the crust is about 10 kilometers thick, increasing to an average of 30 kilometers in the continents. The towering topography of the Alps is supported by a crustal root that reaches down to almost 60 kilometers depth. We call the balance of forces supporting the Earth’s surface isostasy. As we will see, disturbing this balance causes the Earth’s surface to rise or fall.
That’s not it
The thick Alpine crust was formed by a continental collision between the Adriatic Plate (the south side of the Alps) and the European Plate (the north side) starting around 34 million years ago. These landmasses were once separated by the Alpine Tethys Ocean. Subduction pulled the two continents towards each other, closing the ocean and causing them to collide. Compressive forces stacked and squished slices of the colliding crust, thickening it, and building high topography. This collision ended around 5 million years ago. So, to explain the uplift today, we need to look for another cause. Let’s just continue our uplifting story with a next clue.
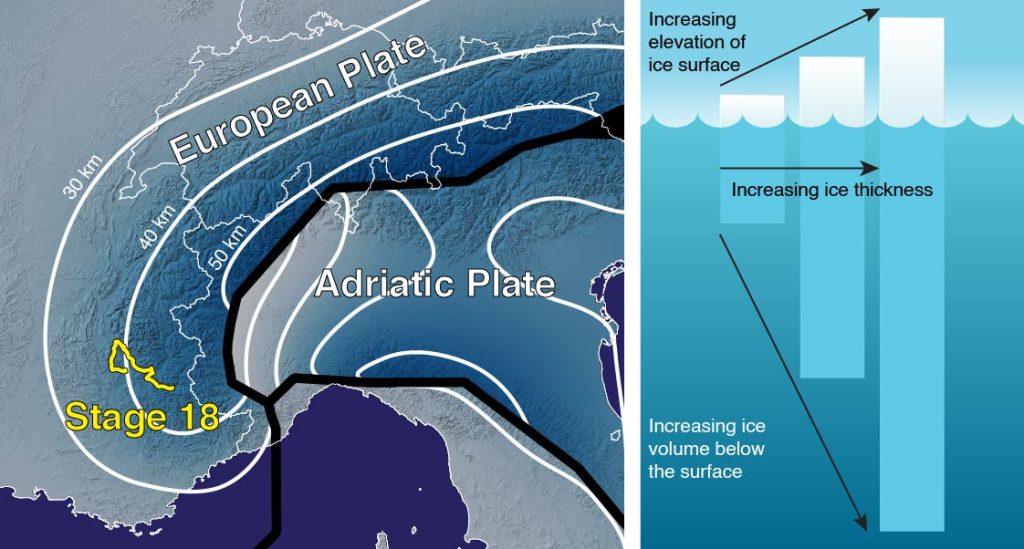
Right: Columns of ice floating in water. Thicker columns of ice support higher elevations. Only the tip of the ice column is emergent. Most of the ice volume is submerged, buoyantly supporting the emergent ice.
But then, what is the cause of uplift?
In the last ice age, which peaked around 20 to 23 thousand years ago, the Alps were covered by a thick ice sheet. The weight of this ice pushed down on the lithosphere, depressing the land surface. The ice load was released when the ice sheet rapidly melted, and the land surface began to rise and recover to its pre-glacial elevation. Initially, uplift rates of ten millimetres a year may have affected the Alps. Thousands of years later, we still witness the effects of that unloading, but at rates of around one millimeter a year. So this explains half of the present-day uplifting of the Western Alps. Geoscientists think (Sternai et al.) the remaining half relates to the dramatic death throes of subduction below the mountain belt.
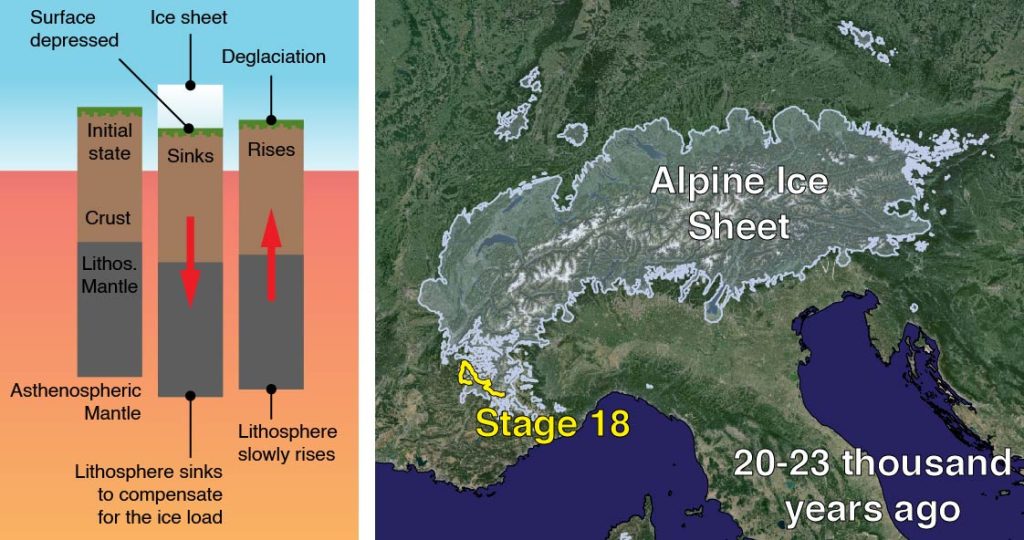
Right: The extent of the Alpine Ice Sheet at the last glacial maximum, 20 – 23 thousand years ago, was mapped by observing landforms left by the ice. Stage 18 of the Tour de France is located at the edge of the former ice sheet. The ice sheet boundary is based on Ehlers & Gibbard.
Death of a subduction zone
Subduction, which ultimately caused the collision of the European and Adriatic continents, was caused by the pull of the dense, heavy oceanic lithosphere sinking into the asthenospheric mantle. This subducted lithosphere—called a slab—was a heavy load, pulling down on the Alps above.
In active subduction zones, the slab leaves a trail of deep earthquakes caused by the stresses of subduction. Geophysicists also use seismic waves travelling through the Earth to scan the depths below the Alps. It’s a technique called teleseismic tomography. Using these approaches, they find that the slab is no longer connected to the surface.
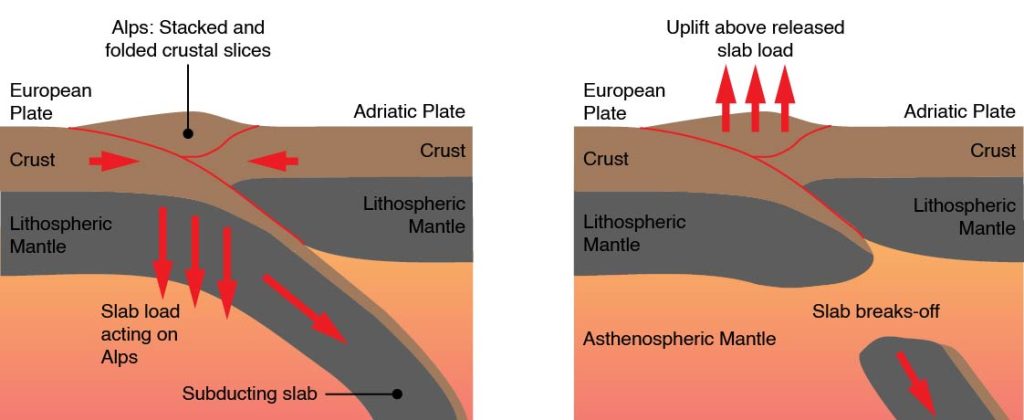
Right: The thick, buoyant European crust resists the slab’s pull, eventually causing it to break off. The release of this slab pull causes the mountain belt to rise.
As the continental collision progressed, the thick, buoyant continental crust of the Alps and European plate resisted the pull of the sinking slab. Eventually, this tension caused the slab to break from the surface. Break-off removed the heavy slab load, causing the Alpine crust to uplift. It was free to rise again.
The subduction zone below the Western Alps may be dead, but the mountains are alive. They are sustained by the transient effects of deglaciation and the slab slipping into the depths of the mantle. By disturbing the isostatic balance of the lithosphere, both processes continue to shape the mountains around Stage 18. That makes this stage a rather uplifting story.
NB: Blogs in other languages than English are all auto-translated. Our writers are not responsible for any language and spelling mistakes.